Researcher Seeks to Unlock DNA Secrets to Combat Deadly Diseases
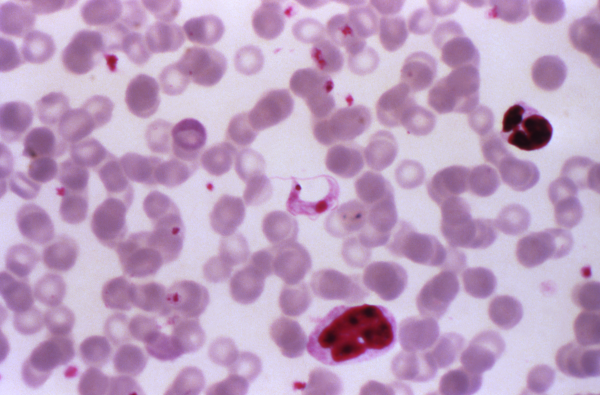
Sleeping sickness, Chagas disease and other devastating illnesses threaten the lives of millions of people and livestock worldwide, particularly in developing Latin American and African countries. While tsetse fly and other insect bites spread the diseases, the true culprits are parasites that so far have defied efforts to combat them.
One reason for the difficulty in dealing with these trypanosomatid parasites is their unique mitochondrial DNA structure, known as kinetoplast DNA. UNC Charlotte mathematician Yuanan Diao is working with researchers across the country to unlock the secrets of the organisms’ DNA.
“When we talk about treating a disease, one approach is to attack its replication process and interrupt it so it cannot reproduce,” said Diao, chair of UNC Charlotte’s Department of Mathematics and Statistics. His research interests include Knot Theory and Geometrical Topology.
“If you understand the DNA of an organism well enough, then you could develop a drug that actually interrupts this process,” he said. “Unfortunately in this case, we still do not understand exactly how this DNA works and how it is replicated. Therefore, we have no idea how to attack it. If there is any hope, understanding it will be the first step. Without understanding, there is no hope.”
The DNA in these organisms is organized into a unique network containing several thousand interlocked short circular DNA chains (called minicircles) and a handful of longer circular DNA chains (called maxicircles). The kDNA is confined within a cyclinder, called the kinetoplast disk. In that disk, the DNA concentration is comparable to that of the bacterial nucleoid. While scientists have been able to gain some knowledge about the networks, their function and origin are still largely unknown.
Biologists have questioned whether the interlocking circles connect for a reason, or randomly, and the mathematical models Diao has developed help researchers to study this question.
“The numerical evidence we have obtained indicates that, when you have a lot of the minicircles crowded in a confined space like the kDNA disk, it is natural for them to form a complicated network through linking between adjacent minicircles,” Diao said. “So, the kDNA probably doesn’t really need any other special mechanism for the minicircles to form a complicated network as having been observed by us.”
When these results are interpreted in the context of the mitochondrial DNA of the trypanosome, they suggest that confinement plays a key role on the formation of the linked network, that is, the mere fact that there are too many minicircles crowded in a small space would lead to the formation of a complicated network.
Diao has drawn upon work he did 20 years ago, which he published in an academic paper at that time. That paper considered from a pure mathematical perspective how circles contained in a tight space would interact with each through topological linking. A colleague, Javier Arsuaga of the University of California at Davis, realized the potential connection of that paper and the minicircle network problem in kDNA and approached Diao about collaborating.
They have published academic papers together, including “The effect of volume exclusion on the formation of DNA minicircle networks: implications to kinetoplast DNA,” in the Journal of Physics, along with colleagues K. Hinson and Y. Sun of UNC Charlotte and “Orientation of DNA minicircles balances density and topological complexity in kinetoplast DNA,” in the journal PLOS ONE, along with Victor Rodriguez of Columbia University and Michele Klingbeil of University of Massachusetts.
“Understanding the kDNA network structure is a huge deal,” Diao said. “From a purely mathematical point of view, our research does not produce very deep theorems. However, we are making progress in understanding the kDNA and our work can have a significant impact.”
Words: Lynn Roberson | Image: Centers for Disease Control and Prevention, Dr. Mae Melvin